Mapping Resilience in Nature to Energy [1.3]
Can we map insights from biology (a very complex system) to our energy systems (which are also very complex)?
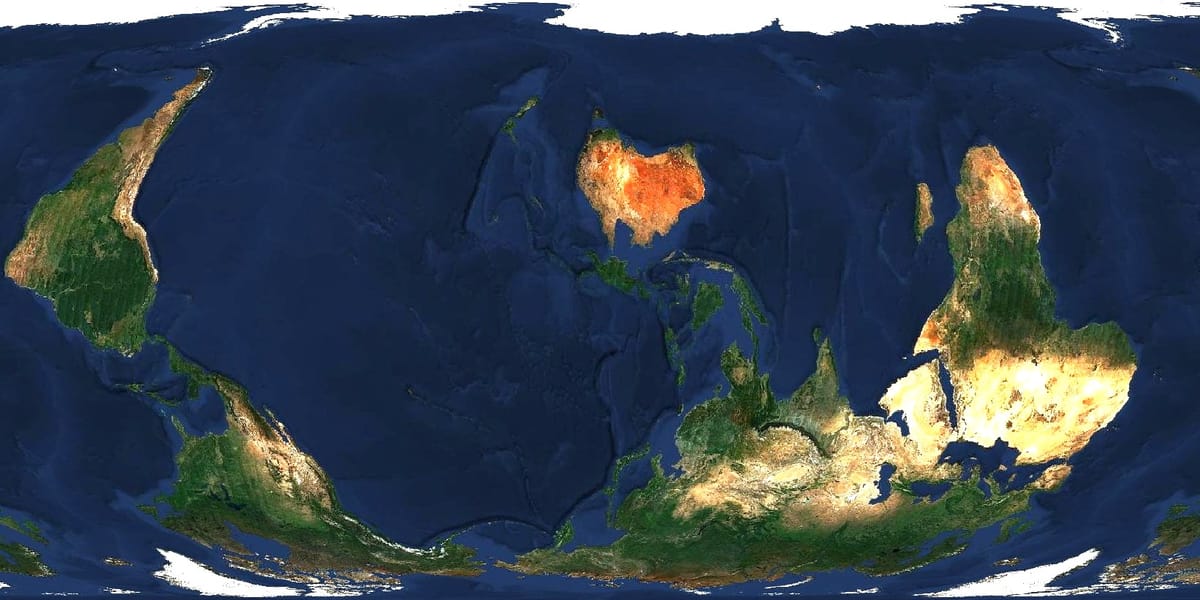
[Links to: summary, part 1.1, and part 1.2]
From last instalment we saw from ant colonies, the boreal forest and photosynthesis that natural selection does not favour the survival of the efficient.[1]
It favours the survival of the fittest – the species and structures most resilient to the world’s chaos and calamities. In these cases and surely others, the species and structures most efficient under optimal conditions get outcompeted when conditions sour, as conditions always eventually do. Efficiency can bring benefits, but to chase efficiency is to fight Darwin. Resilience is more foundational.
So, can we map these insights from biology (a very complex system) to our energy systems (which are also very complex)?
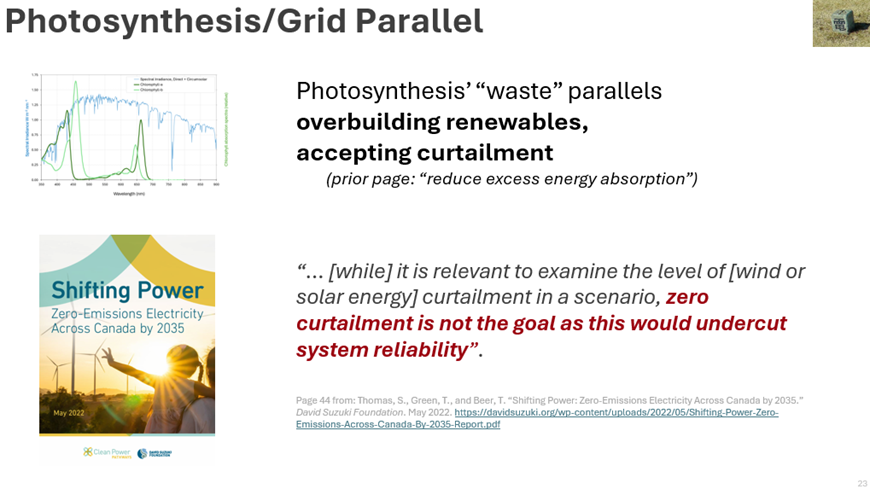
Through the lens of the nature, a resilient human energy system might include significantly overbuilt wind and solar energy resources, tolerating that at times of high production some would be wasted through curtailment. Rather than to try to make use of every electron, some proportion of the wind and solar energy harvest might simply go ignored. This would parallel how photosynthesis has evolved to reduce excess energy adsorption, as noted by Kume last chapter.
Many, probably most, net zero energy models involve significantly overbuilt renewables capacity; from last chapter we can infer that these could be very resilient systems – nature-based solutions, to borrow the vocabulary of the David Suzuki Foundation. To quote from one of its recent reports: [2]
“… [while] it is relevant to examine the level of [wind or solar energy] curtailment in a scenario, zero curtailment is not the goal as this would undercut system reliability”. (Emphasis added by this author.)
The zero-emissions electricity system the David Suzuki Foundation has modelled optimizes for reliability (for general purposes we can substitute the term resilience) instead of optimizing for efficiency, which would amount to zero wind or solar curtailment. Here again, resilience was more foundational.
Staying with electric power generation, that sector has also historically included ample low utilization or low capacity factor generation assets that spend most of the year unemployed.
Canada’s large, clean, dispatchable hydroelectricity-dominated grid is unusual among advanced nations, but in the United States “peaker plants” – most of them powered by natural gas – have historically been used to meet electricity demand at times of peak demand. A 2024 study by the U.S. Government Accountability Office identified that in 2021, peaker plants in that country represented 19 percent of installed generation capacity, but only 3.1 percent of overall electricity production.[3] Here we may find a parallel to the lazy ants: a reserve workforce only ever called into service in times of urgency.
The capacity factor of that U.S. peaker plant fleet in 2021 was a bit above 6 percent.[4] That is to say, if these were run at full power when in use, they would have been inactive 94 percent of the year. That capacity factor would be a challenging datapoint for an efficiency-first worldview, but it aligns with what one would expect in a resilience-first world: adaptive capacity made possible by inefficiency and inventory to maintain uninterrupted electricity production. (It should be noted that peaker plants operated during summer electric demand peaks are broadly being outcompeted by solar panels and battery storage.)
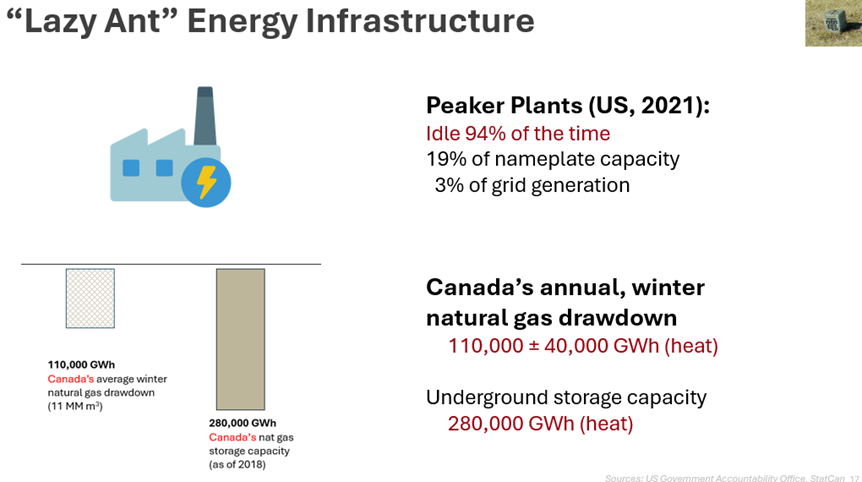
The past and present of our electric grids have always included low-capacity factor assets which sits inactive most of the time, so a future grid dominated by intermittent wind and variable solar assets that also sit inactive most of the time, isn’t intrinsically new. Nor would it be intrinsically new if sometimes they sat inactive when the wind blew or sun shone, if there was no grid demand.[5] Historically, not every summer peaker plant would have been put into operation every summer midday/evening.
The main difference is that we have historically used a fleet of dispatchable power plants which could be turned on or off at will; in Canada this has mostly been served by hydroelectricity. Wind and solar don’t have that flexibility, so in addition to other solutions we may also build some new (potentially infrequently used) dispatchable power plants to maintain the robustness of the rapidly growing electric grid we’ve come to expect and rely on.[6]
And in fact recent concerns about the stability of North American power grids relate in part to "baseload" dispatchable power plants being retired without enough new dispatchable power plants being built to replace them. The regulators want more dispatchable power plants built because/in case they'll be needed at some point – like an ant colony making sure there are plenty of lazy ants to go around, even if they don't do much, most of the time.
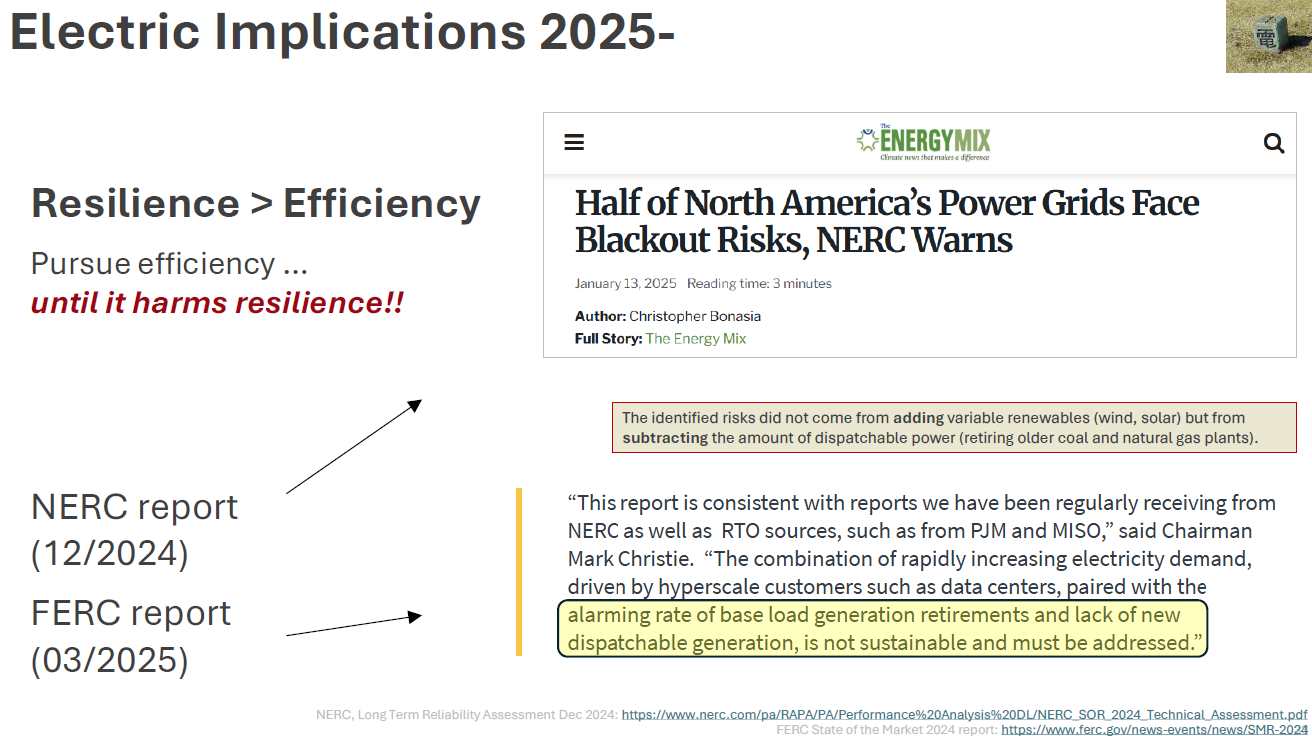
Conifers’ counterintuitive dominance in the boreal forest over broadleaf trees (and their leaves’ radically superior ability to harvest sunlight) can also inform our approach to resilient Canadian energy systems. Broadleaf trees’ radical efficiency helps them dominate temperate and tropical forests; they are the fittest competitors in these biomes, where moisture loss, snow accumulation and other factors are lesser concerns – but they are not the fittest competitors in the boreal forest. (Rising temperatures many change this dynamic.)
Broadleaf trees and conifers are a suitable analogy for electric heat pumps and gas heating respectively. In a context without nuclear and renewables siting concerns, and free of electric transmission and distribution constraints – a context which may describe parts of the world – we may expect electric heat services to dominate.
Unfortunately, that Cinderella’s slipper does not fit Canada’s foot. As shall be discussed in future chapters, Canada draws down its underground natural gas reserves by roughly 110,000 GWh (heat basis) each winter. And as compared to the burly grid interconnections between European nation-states, some of Canada’s interprovincial transmission interconnections are minuscule.
We can encourage the electrification of energy services, which bring many benefits. It would still be incautious to assume Canada can and will build a best-case electric grid, transforming everything everywhere at once: radically expanding electric transmission, resolving every node of grid congestion, hardening infrastructure[7] and driving to 100 percent on consumer preference (another upcoming topic).
Parallel gas energy infrastructure would provide more adaptive capacity. Just as conifers “counterintuitively” dominate the boreal forest, gas heat may be “counterintuitively” prominent in Canada’s boreal context – at least to observers looking through the lens of efficiency, and not the lens of nature.
[Think this might interest a friend or colleague? Forward it their way! Coming soon, Resilience in Business.]
[1] To avoid the necessity of evaluating every species, we might more precisely say that nature does not always favour the efficient.
[2] See page 44 from: Thomas, S., Green, T., and Beer, T. “Shifting Power: Zero-Emissions Electricity Across Canada by 2035.” David Suzuki Foundation. May 2022. https://davidsuzuki.org/wp-content/uploads/2022/05/Shifting-Power-Zero-Emissions-Across-Canada-By-2035-Report.pdf
[3] U.S. Government Accountability Office, Electricity: Information on Peak Demand Power Plants, GAO-24-106145, 21 May 2024. See page 2. https://www.gao.gov/assets/870/868747.pdf
[4] From Table 2 of the U.S. Government Accountability Office report, 129,046,142 MWh of electricity was generated from 237,367 MW of nameplate capacity, the equivalent of 544 hours of production at nameplate capacity. With 8,760 calendar hours per year the capacity factor of the US peaker plant fleet was (544/8,760) = 0.062, or 6.2%.
[5] Battery electric storage can allow otherwise curtailed solar and wind to be stored and dispatched at later times; for example, charging batteries with solar power at midday and discharging them in the evening or even overnight. Even so, a resilient renewables-dominated electric grid is more likely to curtail some wind and solar power than to attempt to leave no electron behind.
[6] Harvey, Fiona. “UK may need new gas-fired power stations to decarbonize grid.” The Guardian. 23 July 2024. https://www.theguardian.com/environment/article/2024/jul/23/uk-may-need-new-gas-fired-power-stations-to-decarbonise-grid.
[7] For example, given how much drone technology is likely to advance by 2050, putting destructive power in the hands of even the most marginal fringe of violent actors, would above-ground transmission lines even be feasible to defend?